by Christophe Bosquillon
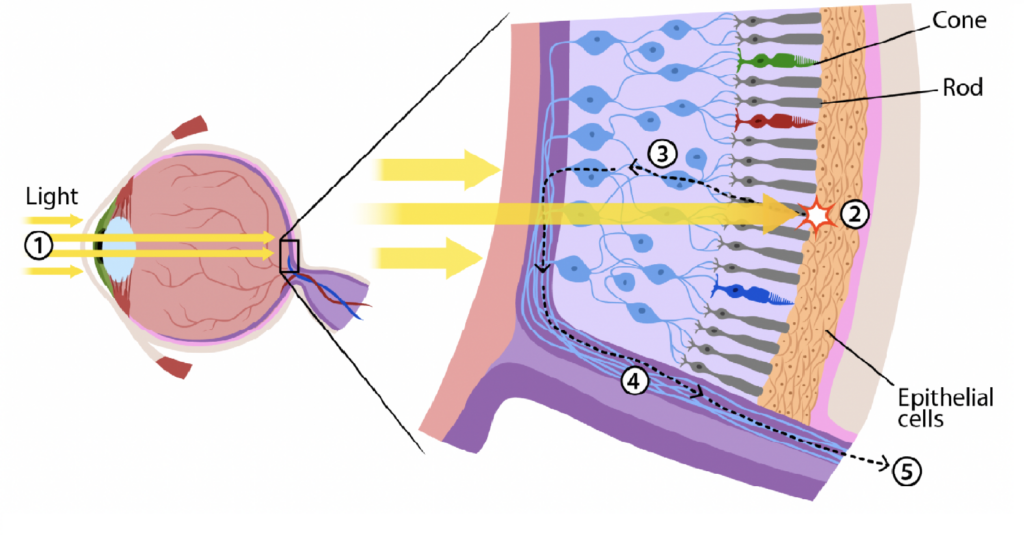
Part 3: LambdaVision and the artificial retina: fundamentals
Blindness due to retina diseases affects over 200 million people globally, and that number is expected to reach 290 million by 2040. The two most common retina degenerative diseases are called Retinitis Pigmentosa and Age-related Macular Degeneration (AMD). Retinitis Pigmentosa affects one and a half million people globally. It starts with peripheral vision that goes dark, until the person is completely blind. AMD affects in the US alone 30 million people over 55, including 10 million in the blinding stage. AMD starts with central vision that goes dark, until the person is totally blind. Solutions originally developed for damaged optic nerves were mainly electronic-based prosthetics (a bionic replacement of the eye), but besides costing $150,000 and requiring invasive brain surgery, they returned a set of gross light and dark pixels from the signal sent through the optic nerve, nothing like natural functional vision. Could biotechnology find a better solution?
Natural vision in a nutshell
Our retina is a thin layer in the back of our eye formed by millions of photoreceptors called cones and rods. During the day, we perceive colours through cones, which are made to see blue, green, and red. At night, the retina switches to rods, giving us this greyish vision typical of night. There are 6 million cones and 120 million rods. These photoreceptor cells, when stimulated by photons, react by sending an electro-chemical signal to other cells located further behind in the retina: first, bipolar cells, then ganglions cells, whose meshed axons form the optic nerve. The signal transmitted to the optic nerve for processing by the brain gives us what we perceive as natural vision.
Can we grow a new retina?
In the past decade, several options to grow a retina have been studied in the US and Japan (RIKEN). Initially, this main biotech approach consisted in trying to reconstruct the natural cones and rods pattern, which seems so difficult that it hasn’t led to a solution that would work. But how about instead trying to replace our natural cones with an artificial but similarly performant photoreceptor pattern? To get to where we need to be, let’s first take a closer look at proteins in the natural retina.
Characteristics and role of proteins in the retina
The building blocks of our retina’s photoreceptors (cones and rods) are a class of proteins called “opsin“. Opsin’s properties come from a substance called retinal, a pigment molecule classified as a chromophore, which means a molecule that absorbs the energy of photons. Located within the cones and rods, the opsin protein generates light-activated vision. “Opsin” comes as “photopsin” in the cones, enabling day vision of blue, green, and red colours. Counter-intuitively though, it is the “rhodopsin“, the protein contained in the rods and suited for night vision, that led to a solution.
Could we find a natural source of protein with properties similar to rhodopsin?
A synthesized photoreceptor protein as a substitute for damaged cones and rods
The main idea beyond a synthetic retina is that instead of trying to reconstruct the original cone and rod pattern, we want to substitute this destroyed mosaic with a new, synthetic layer containing a protein with the same photoreceptor properties. But we don’t know how to produce retinal photopsin nor rhodopsin. And we still need to re-engineer the ability to transmit accurately an appropriate electrochemical signal to the brain via the optic nerve. Knowing that these photoreceptors (120 million rods and 6 million cones) form a mosaic layer on the retina with a 95/5 rods/cones ratio in favour of rhodopsin vs photopsin, we want to look in the direction of rhodopsin first.
The question becomes: could we find a natural source of protein with properties similar to rhodopsin? If we could synthesize and purify that protein, and use it as a building block, we might be able to produce a photoreceptor blanket that transmits an appropriate form of electrochemical signal to the brain via the optic nerve. That blanket would act as a substitute for the destroyed mosaic of cones and rods, resulting in some decent degree of functional vision.
An ancient bacterium meets modern bio-nanotechnology
Three and a half billion years ago, Archaea, a group of single-cell microorganisms, possibly the oldest life form, appeared on Planet Earth. That domain included so-called Halobacteria, and one of these was named the Halobacterium Salinarum, after humans discovered this micro-organism in extremely salty marshlands. From Halobacterium Salinarum you can synthesize a protein called bacteriorhodopsin. Just like the rhodopsin from the natural retina, bacteriorhodopsin also contains retinal. If you could use bacteriorhodopsin to produce an artificial retina, the eye operation would be almost as routine as intervening on a retina “unglued” from the container of the eye, a common occurrence. Then, instead of how many electronic receptors and chips you can pack on a bionic eye, your issue would be how much bacteriorhodopsin you can stuff in an artificial retina to obtain something close to natural functional vision.
Enters LambdaVision
Almost half a century ago Dr. Robert Birge, distinguished chair in chemistry at the University of Connecticut, made his career mission to study bacteriorhodopsin, in order to incorporate light-activated proteins into biomolecular electronic and therapeutic applications, including protein-based artificial retinas.
However, at the time, the principle of protein-based artificial retina was just the beginning. It is not possible to build a fully functional artificial human retina based on the sole property of light-activated proteins. A significant difference between bacteriorhodopsin and human rhodopsin for example is how it converts photon energy into metabolic energy: contrary to a natural retina (where mostly a movement of sodium, potassium, and glutamate ions occur), in the case of bacteriorhodopsin, its hydrogen ions that get pumped across a membrane, creating what is called a proton gradient.
LambdaVision, based in Farmington, Connecticut, USA, was founded in 2009 by its President and CEO Dr. Nicole Wagner and co-founder chemist Dr. Jordan Greco. Dr. Robert Birge is a Member of the Board of Directors of the company, together with several key stakeholders. In this one-hour video featuring Dr. Wagner “New Frontiers: Manufacturing a Protein-Based Artificial Retina in Low Earth Orbit”, the first 40 minutes review the company’s outlook and business.
But how about trying instead to replace our natural cones with an artificial but similarly performant photoreceptor pattern?
The research and manufacturing process adopted by LambdaVision is akin to thin-layer technology. As mentioned above, the deal is to generate a proton gradient that is powerful enough to get to the optic nerve, so that one could gain functional vision with this artificial retina. So, what you need first is a membrane that is permeable to ions. Then on top of that membrane, you’re going to deposit a layer of purified bacteriorhodopsin. It turns out you’re going to need not one, but two hundred of these layers. That number is the minimum necessary to absorb enough light to generate an ion gradient, that in return can sufficiently stimulate the neural circuitry of the bipolar and ganglion cells on the way to the optic nerve, while still fitting within the limited retina size of an eye.
What difference would micro-G make when producing this artificial retina?
The specifics of producing this 200-layer thin film that is the base component for this artificial retina are labelled as a “layer-by-layer electrostatic deposition” process. Practically, that consists in dipping a substrate, like a piece of gauze, in multiple solutions between multiple beakers, and by doing this hundreds of times, you end up with your 200-layer thin film. When you do that in a terrestrial environment, your process is subjected to certain gravity and air-induced constraints, such as sedimentation, evaporation, convection, hydrostatic pressure across your layers, etc. All these factors will affect the uniformity, direction, and homogeneity of the substrate as it develops. Furthermore, with such a thin film, irregularities on one layer are compounded 200 times. That turns into a huge quality control, efficiency, productivity, and ultimately cost problem. And when you know that micro-G removes all these disruptions, it’s a no-brainer that you would want to try it.
Let’s also note that this approach akin to thin film technology is strongly affected by the ability to control fluid-based processes, that are called “fluidics”. This is why it is quite different from the bioprinting equipment available on the ISS and will therefore require its own dedicated hardware. And one major challenge and the reason why these precisely controlled processes matter, is that an extremely precise and uniform orientation of the proteins in the thin film within the artificial retina is a sine qua no condition for functional vision. Without it, you wouldn’t be able to generate an ion gradient across the bipolar and ganglion cells in a strictly uniform direction toward the optic nerve. Signals would go haywire and cancel each other if running in opposite directions.
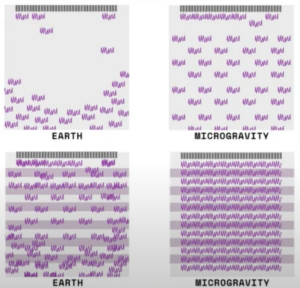
Assessing comparative processes between Earth and orbit
The above fundamentals lead in practice to the manufacturing process of a functional vision device. But what is also required is a measurable comparison with terrestrial properties after the transfer of that process to orbit. First, it is a matter of fulfilling four specifications:
- Highly oriented: the bacteriorhodopsin layering must be precisely oriented for a unidirectional ion gradient to reach the optic nerve without straying.
- Tunable: the layering must meet an optimal density so that it can sufficiently absorb light and stimulate the retina.
- Bio-compatible: the ion-permeable film that supports thin-film layering has previously been used in the ocular space.
- Stable: long term activity will be required from the artificial retina implant, and for that, the condition is high thermal and photochemical stability.
But that’s not all. Once you have built your thin film with 200 layers, you expect it to fulfil four functionalities:
- Effective: the proton gradient has already been proven to stimulate degenerated rat retinas, so yes, it does work on laboratory animals.
- Selective: an unsolicited artificial retina will generate little to no retinal activity, so it is not like the artificial retina would start to “hallucinate” ion gradient-based “vision”.
- Sensitive: contrary to the existing bionic eye with a set of gross clear and dark pixels and no functional vision, the artificial retina based on multi-electrode array experiments demonstrates 200 micro-meter pixel diameter (0.2 millimetres), a satisfactory level of resolution.
- Restorative: the signal transmission speed as tested on lab rats is of 150 milliseconds, compatible with how the human optic nerve and brain normally work. This time-based or temporal resolution is sufficient to support functional vision.
The above constitutes the main menu of specifications and functionalities that is required to conduct comparative experiments and manufacturing operations on Earth and in orbit and compare results. In Part 4 we look at the operationalization of LambdaVision’s research and development as it merged with ISS operations in a process serendipitously initiated in 2016 and executed over 6 missions between 2020 and 2022.
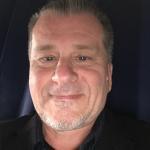
Christophe Bosquillon has a diverse professional background, having operated globally with a focus on the Indo-Pacific region. His experiences in Japan, the Koreas, Taiwan, China, ASEAN, India, Russia, and Australia have given him a deep understanding of the multipolar realpolitik of our world under the Pax Americana. With a background in engineering, trade, and foreign direct investment in industries relevant to Space Resource Utilization (SRU), such as mining, transportation, energy, manufacturing, agrifood, environment, and digitalization, Chris is committed to developing SRU value chains that benefit the Earth. As an executive, owner, writer, and founder of Autonomous Space Futures Ltd, Chris has extensive experience in collaborative policy crafting and works to develop space business and governance models relevant to society. He is a member of NGOs that provide input to the United Nations Committee on the Peaceful Uses of Outer Space (UNCOPUOS) legal subcommittee Working Group on Space Resources. Chris contributes to regulatory clarity on appropriation, priority, sustainability, and sharing in a way that balances national interests with civil society inclusion, provided a transparent due process is followed. When advocating for access to technology and space for the Global South, Chris believes that emerging space powers’ participation in space markets must be commensurate with their interest and involvement in international space politics. He believes that their ability to develop sovereign domestic capabilities with spillover potential is also essential. Chris is keen on ‘Peace Through Strength’ diplomacy and deterrence-based security as enablers of secure space access. He supports sovereign cislunar space situational awareness as mandatory for freedom of circulation in the space domain and deconflicted cooperation on the Moon.